Astronomy has a lot of technical terms you need to learn. Of course, you can always simply step outside on a clear night and look up at the stars, but it always helps to have a deeper understanding of your hobby. Understanding the more technical aspects won’t detract from your enjoyment of the night sky, and can even help you to get more out of it.
For example, you’ve probably already heard of right ascension and declination, and you may already have an idea of what these terms mean, but what do those numbers actually represent? And how are they best used when you’re stargazing?
What is Right Ascension and Declination?
Essentially, the night sky can be thought of as a celestial sphere, like the Earth. However, instead of standing on the sphere, as we do on the Earth, the celestial sphere surrounds the Earth. We’re looking up and outward at the celestial sphere, as though we’re standing within it. As we look up, the Earth turns below our feet and our view of the celestial sphere changes.
When it comes to the Earth, we use latitude and longitude to specify where towns, cities and other landmarks are on the globe. The borders of countries may change, but the towns, cities and landmarks will physically remain where they are.
It’s a similar story when it comes to the night sky. We use right ascension (RA) instead of longitude, and Declination instead of latitude to describe an object’s position on the celestial sphere. Stars and deep sky objects (such as star clusters, nebulae and galaxies) don’t appear to move in our lifetime, so you can think of them as being the celestial equivalent of towns, cities and landmarks on Earth.
However, not everything on Earth is stationary. If you’re using GPS in your car, the GPS satellites triangulate your position to get a precise measurement of your latitude and longitude as it changes. When it comes to the celestial sphere, the Sun, Moon, planets, comets and asteroids all move, just as cars do, so their RA and declination will change too.
That’s the basic concept, but there are, of course, a few differences between Earth-based and celestial navigation.
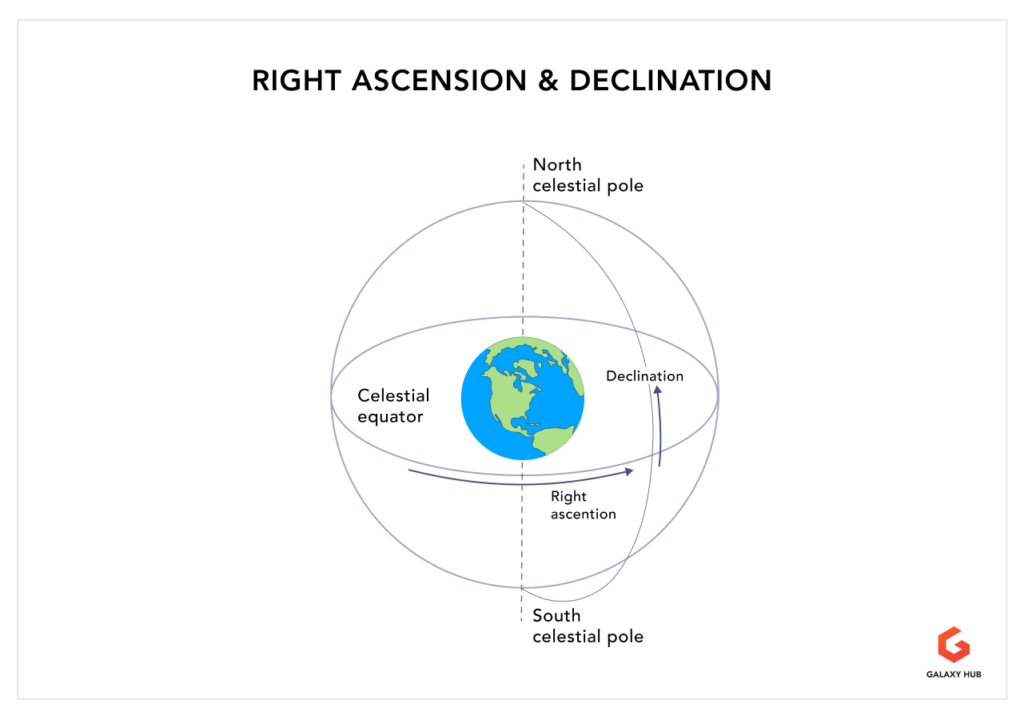
How Right Ascension and Declination Are Different From Latitude and Longitude
Latitude on the Earth and declination on the celestial globe work in essentially the same fashion – both are specified in degrees, ranging from 90 degrees at the most northerly point, 0 degrees at the equator and -90 at the most southerly point. For example, both the north pole on Earth and the northern celestial pole in the night sky are at 90 degrees north.
Right ascension works a little differently.
On Earth, longitude, like latitude, is measured in degrees, but unlike latitude, the range runs from 180 at the most easterly point and -180 at the most westerly point. Zero degrees is defined as being at the Greenwich Observatory in London, England. (If you visit the observatory, you can literally stand with one foot in the eastern hemisphere and one foot in the western hemisphere!)
There are a number of differences between RA and terrestrial longitude. For starters, instead of RA being measured in degrees, it’s measured in hours, minutes and seconds, from zero to twenty-four. It also begins where the path of the Sun crosses the celestial equator from the southern to the northern celestial hemisphere.
Want to get started in astronomy?
Our free telescope cheat sheet breaks down the key factors to choosing a telescope and shows you how to get stunning views of planets, nebula, and galaxies!
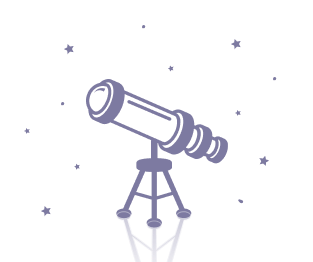
Why is Right Ascension Measured in Hours, Minutes and Seconds?
Another key difference is that there’s no eastern or western hemisphere, as an object’s RA position could range from 0 hours 0 minutes and 0 seconds to 23 hours 59 minutes and 59 seconds. (Or as close to 24 hours as you can precisely measure.) No object has an RA of 24 hours 0 minutes 0 seconds, as once RA reaches 24, it resets to 0 again.
The reason for this is fairly simple: it’s convenient. Roughly speaking, a day on Earth lasts 24 hours, which is the amount of time it takes for the Earth to spin once on its axis. The sky therefore appears to rotate through 15 degrees in a single hour (360 degrees divided by 24 is 15).
As mentioned earlier, one thing to remember is that the stars and deep sky objects will keep the same RA and declination, as they don’t appear to visibly move from night to night. However, the Sun, Moon, planets, comets and asteroids all move (even if it’s only a little) and so their RA and declination will be different from one night to the next.
Why Use Celestial Coordinates?
While astronomers have been observing the night sky for thousands of years, it wasn’t until the invention of the telescope that a coordinate system was properly developed. Until that point, if you wanted to describe the location of an object to a fellow astronomer, you needed to provide instructions using a recognized star or constellation as a starting point.
If you were observing with the naked eye, this was pretty straightforward, as the object you were looking for would also be visible to the naked eye. For example, if you wanted to tell someone how to find the Orion Nebula, you only had to tell them to look just below the three stars of Orion’s belt.
(Bear in mind that the 88 constellations, as we know them today, weren’t formalized until 1928. Prior to that, there were many faint constellations invented that were not widely known – so one astronomer could say an object was within a degree of the brightest star in the constellation Felix, but others may not be familiar with that constellation!)
Things changed with the invention of the telescope, as fainter objects were being discovered that would need to be observed and studied by others. Some were relatively easy to find; for example, M57, the Ring Nebula, lies between the stars Beta and Gamma Lyrae, while M35 lies close to Mu and Eta Geminorum.
However, try describing how to find M3, which lies in a fairly barren area of the sky between Arcturus in Bootes and Cor Caroli in Canes Venatici. That particular cluster lies at least 12 degrees away from either star, with no other bright star less than ten degrees away.
Creating a coordinate system allowed astronomers to precisely specify the location of an object, which then allowed other astronomers to easily find that same object on a star chart. This was particularly important when it came to new discoveries, and remains a vital part of the science today – especially when it comes to comets, which can move quickly over the course of several nights.
Solstices, Equinoxes & Precession
Everyone knows that there are four seasons – spring, summer, autumn and winter – but how do we know when each season officially begins? You’ve probably heard of solstices and equinoxes, but what are they?
While the weather might have other plans, the official start of each season is determined by the position of the Sun on the celestial sphere, as determined by its RA and declination, particularly with relation to the celestial equator.
As with the terrestrial equator on Earth, the celestial equator marks a declination of 0 degrees on the celestial sphere. Theoretically, with the possible exception of any location very close to the poles, any object with a declination of 0 degrees is visible from almost anywhere on Earth.
How the Seasons Are Determined
How does this relate to the seasons? Each season begins when the Sun either crosses the celestial equator or is at its highest point above the celestial equator.
More specifically, in the northern hemisphere, the seasons change as follows:
Spring begins when the Sun crosses the celestial equator from the southern to the northern celestial hemisphere. At that point, it has a declination of 0.0 degrees.
Summer begins when the Sun reaches its highest point in the northern celestial hemisphere. At that point, it has a declination of 23 degrees and 26 minutes above the celestial equator.
Autumn begins when the Sun crosses the celestial equator from the northern to the southern celestial hemisphere. At that point, as with the start of spring, it has a declination of 0.0 degrees.
Winter begins when the Sun reaches its lowest point in the southern celestial hemisphere. At that point, it has a declination of minus 23 degrees and 26 minutes below the celestial equator.
In the southern hemisphere, the seasons are the opposite of the seasons in the northern hemisphere. So instead of spring, those in the southern hemisphere experience autumn, and instead of summer, the southern hemisphere has winter.
Solstices and Equinoxes
We have summer and winter because the Earth’s axis is tilted. In June, the northern hemisphere of the Earth is tilted toward the Sun, while the southern hemisphere is tilted away from it. Consequently, it’s summer in the north and winter in the south.
The opposite is true in December. The southern hemisphere is pointed toward the Sun, and it experiences summer, while the northern hemisphere is pointed away from the Sun, giving rise to winter.
Regardless of which hemisphere you’re in, the start of summer and winter is always known as the solstice. The word solstice is derived from two latin words: Sol, meaning Sun, and sistere, meaning “to stand still.” It’s so-called because the Sun appears to momentarily stop moving at that time before starting to move back again.
For example, at the start of spring, the Sun appears to rise in the east and set in the west. In the northern hemisphere, the Sun will then appear to rise and set further toward the north, so that it then typically rises in the northeast and sets in the northwest by the start of summer.
It’ll briefly remain stationary around the solstice and then start to move back again. It rises in the east and sets in the west at the start of autumn and then continues its trek further south until the start of winter. On that solstice, it rises in the southeast and sets in the southwest, and briefly remains that way before starting to move toward the north again.
The word equinox is derived from the words aequi, meaning “equal” and nox, meaning “night.” On the spring and autumn equinoxes, the orbit of the Earth causes it to be upright, rather than tilted. As a result, every location on Earth experiences a day and night of equal length.
Precession and the Movement of the Stars
While it may seem as though the stars and deep sky objects are fixed and never move, that’s not strictly speaking true. Whether it’s the Earth, Moon, Sun, planets, stars or even galaxies, everything is moving through space, but this is barely noticeable from our perspective. On a night-to-night basis, the stars appear in the same patterns and rise and set as they always do, but those patterns will change over the course of thousands of years as the stars slowly move.
To make matters worse, we also need to take into account precession. Both the Sun and the Moon exert a gravitational influence over the Earth, which, over time, causes the Earth’s axis to wobble slightly, like a spinning top. One “wobble” takes 25,771 years to complete, during which time, the Earth’s poles will draw a circle in the sky and point toward a different star in the process.
Right now, the closest bright star to the north celestial pole is Polaris in Ursa Minor, but 3,000 years ago it was neighboring Kochab, aka Beta Ursae Minoris. Other past and future bright pole stars include Deneb in Cygnus and Vega in Lyra, but neither of these will come within five degrees of the north celestial pole. Polaris itself will be closest in the year 2100, with only Thuban (Alpha Draconis) coming closer, some 21,000 years from now.
As a combined result of movement and precession, the positions of everything in the sky will eventually change, and their RA and declination will need to be updated. For astronomers, who frequently rely on accurate measurements, the RA and declination of stars and deep sky objects are updated every 50 years. This happened in the year 2000 and will happen again in 2050. As we draw closer to that date you’ll see more and more catalogs listing the positions for that time (known as the epoch.)
Incidentally, while there are no bright stars that are currently close to the southern celestial pole, Sirius, the brightest star in the sky, will one day be less than two degrees away. This is due to a combination of the star’s movement through space and precession. Unfortunately, if you want to see this for yourself, you’ll need to wait another 64,000 years!
Want to get started in astronomy?
Our free telescope cheat sheet breaks down the key factors to choosing a telescope and shows you how to get stunning views of planets, nebula, and galaxies!
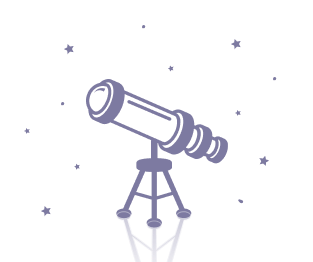
Do You Really Need to Know Right Ascension & Declination?
As with everything, the short answer here is both yes and no. To some extent, with the advent of computer software and cell phone apps, the need for traditional star atlases has diminished. Amateur astronomers can simply search for an object and then print off a chart showing its position, without ever needing to know its coordinates.
Many astronomers will also use computerized GoTo telescopes, which also tend to negate the need for star charts. After searching the database for your target, the telescope will then automatically turn and point in the right direction.
If nothing else, you should always be aware of an object’s declination, as this will dictate whether it is visible from your latitude. For example, there are a multitude of wonderful star clusters visible from the southern hemisphere, whose declination is too far south to be visible to observers north of Earth’s equator (see below.)
Tips & Tricks
Despite today’s technological society, it’s highly unlikely that right ascension and declination will ever disappear completely, and it’s worth having a core understanding of both to get the most out of your hobby. With that in mind, here are a few tips ‘n tricks that might help.
- Zero-point (an RA of 0 hours, 0 minutes and 0 seconds) is due south at local midnight (or 1AM, daylight savings time) on September 21st.
- Since right ascension ranges from 0 to 24 hours, one month of time equates to 2 hours of right ascension. An object with an RA of 22 hours will be south at local midnight around August 21st, while an object with an RA of 2 hours will be south at local midnight around October 21st.
- To approximately calculate when an object will be due south at midnight, take its RA and divide it by two. This is the number of months after late September that the object will be at its best. For example, if an object has an RA of 10 hours, it’ll be due south at midnight five months after late September, which will be late February. If an object has an RA of 19 hours, it’ll be due south at midnight 9.5 months after (or 2.5 months before) late September, which will be early July.
- Six hours of RA equates to 3 months of time, so objects with an RA of 6 hours will be due south at local midnight at around December 21st.
- An object with an RA of 12 hours will therefore be due south at local midnight (again, 1AM daylight savings time) around March 21st.
- An object with an RA of 18 hours will therefore be due south at local midnight (again, 1AM daylight savings time) around June 21st.
- All objects with a positive declination (greater than zero) are visible from the northern hemisphere, while all objects with a negative declination (below zero) are visible from the southern hemisphere.
- You can easily calculate if an object is visible from your location. If the object has a positive declination, deduct 90 and this will tell you the maximum latitude in the southern hemisphere from which the object should be visible. Conversely, if the object has a negative declination, add 90 to give you the maximum latitude in the northern hemisphere from which the object should be visible. Bear in mind that its actual visibility will depend upon your local conditions (ie, obstructions on your horizon is, light pollution and the thickness of the air close to the horizon.)